GC-MS analyses
Applications
Through GC-MS, the great variety of compounds present in oils and other bitumen, particularly the biomarkers that can be traced back to biochemical in living organisms, can provide a wealth of information about:
- kerogen sources
- depositional environment
- maturity
- alteration
- oil-oil and oil-source correlation
- age indications of oil sources
- migration distance
However, the very complexity of mixtures and wide range of component abundances creates identification and quantification problems, which require the specificity and sensitivity of mass spectrometers to overcome. The specificity and sensitivity arise from the creation of fragment ions from useful molecules that are characteristic of its structure, and monitoring just those ions.
Just a few ions can be used to monitor a wide range of compounds on the basis of their mass:charge ratios (m/z), such as steranes by m/z 217. This operation mode is often termed selected ion recording (SIR).
There are many types of mass analysers, but the most common in petroleum geochemistry are low resolution (LR) quadrupole and high resolution (HR) magnetic sector instruments. Low resolution machines can resolve ions differing by a minimum of one whole mass unit, whereas high resolution instruments can differentiate between fractions of a mass unit. An advantage of accurate mass instruments is that they can analyse total hydrocarbons (i.e. combined saturates and aromatics) because of their great specificity.
For example, 4-methylsteranes (m/z 231.211) can be distinguished from triaromatic steroids (m/z 231.117) and C-ring monoaromatic steroids (m/z 253.196) from n-alkanes (m/z 253.290). A disadvantage is that magnets cannot scan a wide mass range rapidly during SIR analysis and the upper mass should not be more than ~1.5 times the lower. This limits the range of compound groups that can be monitored during a single analysis.
Sometimes two mass spectrometers are coupled in tandem to provide even greater specificity, so the technique is termed GC-MS-MS. A particular use of this method is the creation of parent (molecular) ions for a particular compound type, which are allowed to pass through the first detector assembly, and then decay into specific daughter fragment ions in an intermediate field-free region (often with the aid of an inert collision gas such as argon), which in turn are selectively monitored by the second analyser.
This more complex analysis provides even greater specificity than SIR, but cannot accommodate as wide range of compounds as SIR without sacrificing sensitivity, because of the number of transitions that would need to be monitored. For example, the C27–C29 steranes that can be monitored via m/z 217 by SIR require the three transitions m/z 400®217, 386®217 and 372®217 by MS-MS. Not all of these steranes are fully resolved by GC-MS-SIR, so MS-MS can be required, and particularly for less abundant components that tend to co-elute with other, more abundant, biomarkers. GC-MS-MS can be performed on HR as well as LR instruments, although the same restrictions on m/z range apply as for SIR on HR instruments.
Just because a compound cannot be seen during GC-MS-SIR does not mean it has no influence on an analysis. Large amounts of another compound (e.g. n-alkane) co-eluting with a biomarker can cause signal suppression and slightly alter the relative retention time of the compound of interest. It can be preferable to remove n-alkanes from the saturates fraction prior to GC-MS (yielding a branched/cyclics or b/c fraction) to provide cleaner data on biomarkers. It also permits all acyclic isoprenoids to be monitored by SIR, avoiding co-elution problems of some of the most useful members (head-to-head linked of >C35). However, a total saturates fraction should be used if information from compounds that contain long n-alkyl chains is required, e.g. n-alkylcyclohexanes, as such compounds also tend to be depleted (at least the longer chain members) by all known methods that remove n-alkanes.
So the choice of MS analysis depends upon the requirements of the study and the families of compounds likely to provide useful information. APT offers all of the options described above. For routine monitoring, a minimum set of fragments ions are recommended, as required for all Norwegian studies to be reported to the NPD. Other options are available for more detailed investigations:
- GC-MS-SIR of separate saturates and aromatics by LR-MS or HR-MS
- GC-MS-SIR of combined saturates & aromatics by HR-MS
- GC-MS-SIR of diamondoids (HR-MS or LR-MS)
- GC-MS-SIR of alkylbenzenes (generally LR-MS)
- GC-MS-MS of saturates or b/c saturates by LR-MS or HR-MS
- GC-MS-SIR of carbazoles (HR-MS)
Because the chemical names of biomarkers are often long, it is more convenient to use abbreviations to label chromatograms and in describing various ratios. APT uses abbreviations designated by the Norwegian Industry Guide to Organic Geochemical Analyses (NIGOGA, Weiss et al. 2000).
A general background to GC-MS and the application of the data obtained from its use can be found in Peters et al. (2005), and a basic background to molecular palaeontology in Killops & Killops (2005).
Routine GC-MS-SIR
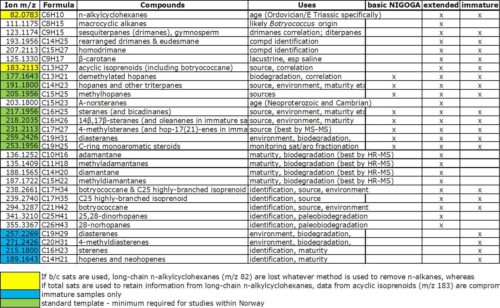
This table shows the accurate masses of the fragment ions APT records during SIR of saturates fractions. The yellow picks out those ions that are affected by whether n-alkanes are removed or not. The green labels indicate the basic set of ions monitored for Norwegian studies (as described in the NIGOGA guide). The blue ions are only worth considering for immature source rock samples.
Three main options are offered by APT, although other ions can be added according to specific requirements.
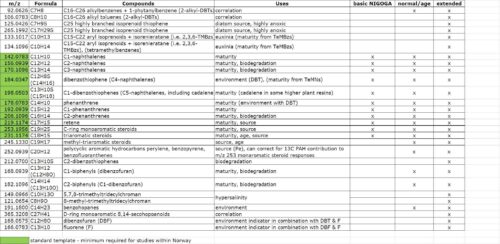
The basic NIGOGA set is combined with the related aromatics shown in this table, and is usually run on the HR magnetic sector instrument, either as individual saturates and aromatics, or as a combined hydrocarbons fraction. The wider m/z range covered by the extended and immature sets requires the LR quadrupole instrument.
The aromatics SIR options are listed in the above table. Again, the basic NIGOGA set, the minimum required for Norwegian studies, is shown by the green highlighting of the accurate masses. The HR instrument is usually used for these analyses, either as a separate aromatics fraction or a combined total hydrocarbons fraction. Other options, spanning a greater m/z range, once again require the use of the LR instrument.
Where two formulae are shown, they share the same nominal mass, so both are monitored via LR-MS by means of the same unitary m/z value. However, when HR-MS is used, only the first named compound group, represented by the single accurate mass in the m/z column, is determined. The other compound group (shown in parentheses) is not monitored because it has a different accurate mass (not shown).
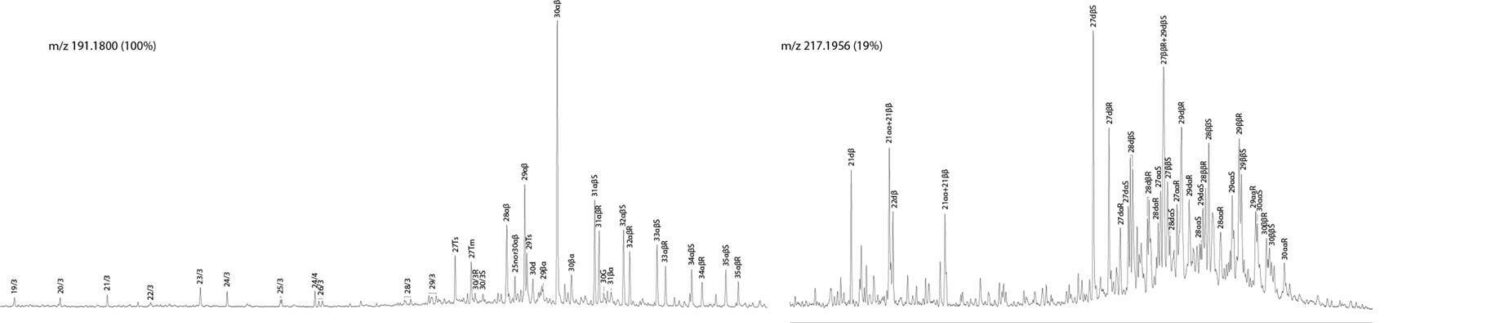
Terpane (m/z 191) and sterane (m/z 217) chromatograms from GC-HRMS analysis of combined saturates and aromatics from NSO-1 oil (retention time axes aligned)
GC-MS-SIR of diamondoids
The only major compound group for which HR-GC-MS-SIR analysis of total hydrocarbons does not work is the diamondoids, which share identical chemical formulae to some aromatics, and so a separate saturates fraction has to be analysed (on an HR or LR instrument). Diamondoids are particularly thermally stable and resistant towards biodegradation, so are useful for assessing advanced thermal alteration and/or biodegradation of oils, and for providing means to correlate such oils (Fang et al. 2013; Moldowan et al. 2015).
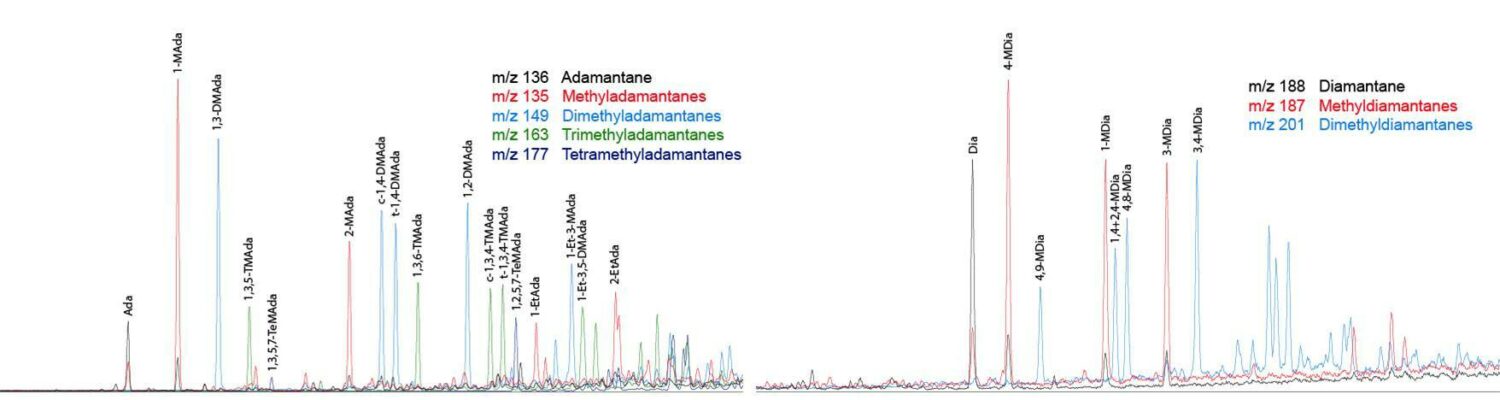
Example GCMS of diamondoids
GC-MS-SIR of alkylbenzenes & benzocarbazoles
Alkylbenzene distributions are mostly used to evaluate reservoir segregation, and benzocarbazoles are used to estimate secondary migration distances, so are considered an aspect of reservoir filling history. Both aspects are considered in the reservoir evaluation section.
GC-MS-MS of saturates
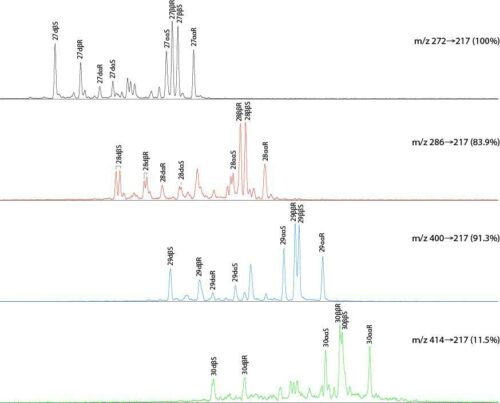
For some GC-MS analyses it can be necessary, or at least useful, to remove n-alkanes from the saturates fraction. For example, it is necessary where distributions of C20+ acyclic isoprenoids are required, because of the unavoidable co-elution of some components with n-alkanes.
It is useful where n-alkane abundance is relatively high to avoid chromatographic overloading when obtaining a reasonable signal from biomarkers, or possible signal suppression where co-elution with n-alkanes occurs on quadrupole instruments.
Sample requirements
Bearing in mind the potential requirement for any subsequent GC, GC-MS or isotopic analyses, 100–200 g of unwashed cuttings (minimum of 50 g) or 20–30 g of washed cuttings, core or outcrop (5–10 g minimum) are required.
Samples are generally selected on the basis of representation of source rock or reservoir intervals, and often on the basis of TOC/Rock-Eval screening of source rocks. All rock samples require milling prior to extraction. Unwashed cuttings will require an initial washing, and a detergent based system is likely to be required where oil based mud is present.
Analytical procedure
HR-GC-MS of saturated and aromatic fractions
For HR-MS analysis a Micromass ProSpec or Thermo Scientific DFS high resolution instrument is used. The instrument is tuned to a resolution of 3000 and data are acquired in SIR mode. The GC column is a 60 m x 0.25 mm i.d. CP-Sil-5 CB-MS (0.25 mm film thickness). The temperature programme is 50°C (1 min.) to 120°C at 20°/min and then to 320°C (20 min. hold) at 2°C/min.
D4-27ααR (i.e. D4-cholestane) is used as internal standard when quantitative results are requested for the saturated compounds. D8-naphthalene, D10-biphenyl, D10-phenanthrene and D12-chrysene are used as internal standards when quantitative results are required for the aromatic compounds. The aromatic and aliphatic (saturates) fractions may be analysed together or separately in HR mode.
LR-GC-MS of saturated and aromatic fractions
For LR-MS analysis a Thermo Scientific TSQ Quantum XLS instrument is used. The instrument is tuned to a resolution of <0.3 mass units and data are acquired in SIR mode. The GC column is a 60 m x 0.25 mm i.d. CP-Sil-5 CB-MS (0.25 mm film thickness), and the temperature programme 50°C (1 min.) to 115°C at 20°/min and then to 325°C (30 min. hold) at 2°/min.
D4-27ααR sterane is used as internal standard in saturates fractions when quantitative results are requested, and for aromatic fractions the internal standards are D8-naphthalene, D10-biphenyl, D10-phenanthrene and D12-chrysene. The saturates and aromatics fractions have to be analysed separately to avoid interference between some compound types. With regard to saturates, either a total saturates fraction or a branched/cyclics fraction (i.e. from which n-alkanes have been removed) can be analysed, according to the compound groups of most interest.
GC-MS-MS of saturates
A Thermo Scientific TSQ Quantum XLS instrument is used, tuned to a resolution of 0.7 mass units. A collision energy of 25 V is used with argon as the collision gas at a pressure of 0.3 mTorr. The GC column used is a 60 m x 0.25 mm CP-Sil-5 CB-MS (film thickness 0.25 µm). The temperature programme is 50°C (1 min.) to 320°C (20 min. hold) at 2.5°/min. D4-27ααR sterane is used as internal standard in saturates fractions when quantitative results are requested.
GC-MS of diamondoids
A Thermo Scientific TSQ Quantum XLS instrument is used to analyse diamondoids in saturates fractions. The instrument is tuned to a resolution of <0.3 mass units and data is acquired in SIR mode. The GC is fitted with a 60 m x 0.25 mm i.d. CP-Sil-5 CB-MS column (0.25 mm film thickness). D16-adamantane is used as internal standard when quantitative results are required (and a correction factor of ~0.5 is applied to diamantane responses). The temperature programme is 50°C (1 min.) to 70°C at 20°C/min., then to 170°C at 1.5°C/min., and finally to 325°C (20 min.) at 20°C/min.
Potential problems
If an oil-based mud (OBM) has been used when drilling, it will certainly influence the GC analysis of the saturated hydrocarbon fraction, typically masking any in-situ components, normally in the C14−C20 (diesel) range. The gasoline and biomarker range hydrocarbons are less affected, although biomarkers may be affected if mud used in a previous discovery well is re-used.
Contamination can occasionally arise from handling of sediment/rock samples where only trace amounts of extractable material are recovered. Among the more common contaminants are plasticisers (particularly phthalates) and squalene.
Because of the complexity of oil and bitumen extracted from source rocks, it is inevitable that there is some degree of co-elution of compounds, no matter what stationary phase is selected. Even the specificity of GC-MS does not obviate the need for checking if automated peak identification and quantification are correct if apparently anomalous data are obtained. For example, in immature rock extracts 17b-hopanes can dominate the m/z 191 mass chromatogram, and some can co-elute with the more usual hopanoids in more mature samples. Similar co-elution can occur between oleanenes and steranes in m/z 217 and 218 mass chromatograms.
Other potential problems are poor chromatography, which can arise from overloading of the column or the gradual increase in contamination of the front end of the column from accumulation of material that does not volatilize adequately (particularly the more polar components from whole-oil analysis). This can lead to poor quantification, because peak shape deteriorates and resolution declines.
It is possible for co-elution to cause signal suppression or enhancement in mass chromatograms. For example, terrestrial oils typically contain particularly abundant hopanes but sparse steranes. On some mass spectrometers this can lead to the most abundant hopanes generating a signal in the m/z 217 mass chromatogram. On other instruments, suppression of signal can be observed (sometimes to below base line) where this type of co-elution occurs.
Other instrument-based variations include changing sensitivity across the mass range, related to tuning (mass calibration and resolution), which can lead to significant variations in ratios based on different ions. One such ratio that can be affected, particularly on low resolution quadrupole instruments, is the hopane to sterane ratio, based on m/z 191 and 217 responses. The routine acquisition of data from standard samples can help identify whether such variations are likely.
Depending upon the concentration of n-alkanes, on rare occasions not all of them may be removed (the smallest are the most difficult to remove). In addition, all methods available for removal of n-alkanes have been found to result in depletion of other compounds with long n-alkyl chains, such as n-alkylcyclohexanes. So if GC-MS analysis of such compounds is required, a total saturates, rather than a branched/cyclics fraction, should be analysed.
References
Fang C., Xiong Y., Li Y., Chen Y., Liu J., Zhang H., Adedosu T.A., Peng P. (2013) The origin and evolution of admantanes and diamantanes in petroleum. Geochimica et Cosmochimica Acta 120, 109–120.
Killops S., Killops V. (2005) Introduction to Organic Geochemisty. Blackwell Publishing, Oxford.
Moldowan J.M., Dahl J., Zinniker D., Barbanti S.M. (2015) Underutilized advanced geochemical technologies for oil and gas exploration and production-1. The diamondoids. Journal of Petroleum Science and Engineering 126, 87–96.
Peters K., Walters C.C., Moldowan J.M. (2005) The Biomarker Guide (vols 1 & 2). Cambridge University Press,
Weiss H.M., Wilhelms A., Mills N., Scotchmer J., Hall P.B., Lind K., Brekke T. (2000) NIGOGA - The Norwegian Industry Guide to Organic Geochemical Analyses, Edition 4.0. 102pp. Available from http://www.npd.no/global/norsk/5-regelverk/tematiske-veiledninger/geochemical-analysis_e.pdf